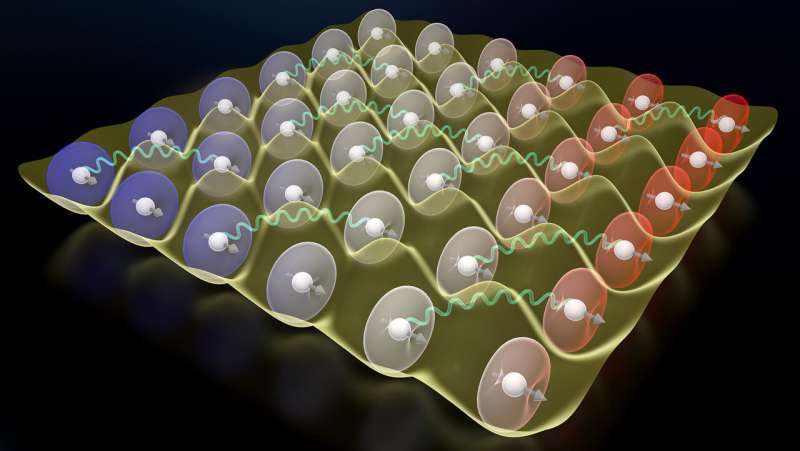
In a
new study published in Science today, JILA and NIST (National
Institute of Standards and Technology) Fellow Jun Ye and his research team have
taken a significant step in understanding the intricate and collective
light-atom interactions within atomic clocks, the most precise clocks in the
universe.
Using a cubic lattice, the researchers measured specific energy shifts within the array of strontium-87 atoms due to dipole-dipole interactions. With a high density of atoms, these mHz-level frequency shifts—known as cooperative Lamb shifts—were spectroscopically studied. These shifts were studied spatially and compared with calculated values using imaging spectroscopy techniques developed in this experiment.
These cooperative Lamb shifts, named because the presence of many identical atoms in a tightly confining space modifies the electromagnetic mode structure around them, are an important factor as the numbers of atoms in clocks continue to grow.
"If you can understand and control these interactions at high density in this grid, you can always make the grid bigger and bigger," explains JILA graduate student William Milner, the paper's second author. "It's an inherently scalable technology, important for improving clock performance."
Time in a cube
Atomic clocks, long regarded as the pinnacle of precision, operate on the principle of measuring the frequency of light absorbed or emitted by atoms. Each tick of these clocks is governed by the oscillations of the quantum superposition of electrons within these atoms, stimulated by the corresponding energy from a probing laser. The laser excites the atoms into a quantum state known as the clock state.
While more traditional optical lattice clocks use a one-dimensional optical lattice, suppressing the atoms' movements only along one strongly confining direction, the strontium quantum gas clock used in this study confined the atoms in all directions by placing them in a cubic arrangement. While using a 3D lattice is an attractive clock geometry, it also requires preparing an ultracold quantum gas of atoms and carefully loading them into the lattice.
"It's more complicated, but it has some unique benefits as the system features more quantum properties," Milner elaborates.
In quantum physics, the spatial arrangement of particles critically influences their behavior. With its uniformity and equilibrium, the cubic lattice created a controlled environment where atomic interactions were observable and manipulable with unprecedented precision.
Watching dipole-dipole interactions
Using the cubic lattice, Ross Hutson (a recent JILA Ph.D.graduate), Milner, and the other researchers in the Ye lab, were able to facilitate and measure the dipole-dipole interactions between the strontium atoms. These shifts, normally so small they are neglected, arise from collective interference between the atoms behaving as dipoles when they are prepared in a superposition of the two clock states.
Because the spatial ordering of the atoms within the cubic lattice influences the dipolar coupling, researchers could amplify or diminish the dipole interactions by manipulating the angle of the clock laser relative to the lattice. Operating at a special angle—the Bragg angle—the researchers expected strong constructive interference and observed a correspondingly larger frequency shift.
Looking at cooperative lamb shifts
With stronger dipole-dipole interactions occurring within the lattice, the researchers found that these interactions created local energy shifts throughout the clock system.
These energy shifts, or cooperative Lamb shifts, are very small effects that are normally hard to detect. When many atoms are grouped, such as in a cubic clock lattice, these shifts become a collective affair and are revealed by the newly achieved clock measurement precision. Left uncontrolled, they can affect the accuracy of atomic clocks.
"These [shifts were] initially proposed back in 2004 as a futuristic thing to worry about [for clock accuracy]," adds Milner. "Now, they're suddenly more relevant [as you add more atoms to the lattice]."
As if measuring these shifts wasn't interesting enough, even more interesting was that the researchers saw that the cooperative Lamb shifts weren't uniform across the lattice, but varied depending on each atom's specific location.
This local variation is significant for clock measurement: it implies that the frequency at which atoms oscillate, and hence the clock's 'ticking,' could slightly differ from one part of the lattice to another. Such spatial dependence of the cooperative Lamb shifts is an important systematic shift to understand as researchers strive to improve timekeeping precision.
"By measuring these shifts and seeing them align with our predicted values, we can calibrate the clock to be more accurate," Milner says.
From their measurements, the team realized there was a close connection between the cooperative Lamb shifts and the propagation direction of the clock probe laser within the lattice. This relationship allowed them to find a specific angle where a "zero crossing" was observed and the sign of the frequency shift transitioned from positive to negative.
"It's a particular quantum state that experiences zero collective Lamb shift (equal superposition of ground state and excited state)," explains JILA graduate student Lingfeng Yan. Playing around with the connection between the laser propagation angle with respect to the cubic lattice and the cooperative Lamb shifts has allowed the researchers to fine-tune the clock further to be more robust against these energy shifts.
Exploring other physics
Beyond controlling and minimizing these dipole-dipole interactions in the cubic lattice, the JILA researchers hope to use these interactions to explore many-body physics in their clock system.
"There's some really interesting physics going on because you have these interacting dipoles," Milner elaborates, "So people, such as Ross Hutson, have ideas for even potentially using these dipole-dipole interactions for spin squeezing [a type of quantum entanglement] to make even better clocks."