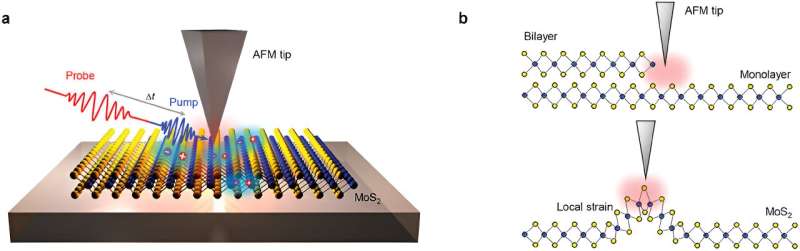
Researchers from the University
of California, Berkeley have developed cutting-edge nanoscale optical imaging
techniques to provide unprecedented insights into the ultrafast carrier
dynamics in advanced materials.
Two studies, published in Advanced Materials and ACS Photonics, showcase significant progress in understanding the carrier behaviors in two-dimensional and phase-change materials, with implications for next-generation electronic and optoelectronic devices.
The research team, led by Prof. Costas P. Grigoropoulos, Dr. Jingang Li, and graduate student Rundi Yang, employed a novel near-field transient nanoscopy technique to probe the behavior of materials at the nanoscale with both high spatial and temporal resolution. This approach overcomes the limitations of traditional optical methods, allowing researchers to directly visualize and analyze phenomena that were previously difficult to observe.
"Our technique enables us to examine how charge carriers and excitons behave and interact at the nanoscale in various materials," explains Li. "This is crucial for understanding and optimizing the performance of advanced devices based on these materials."
In one study, the team focused on atomically thin transition metal dichalcogenides (TMDCs), materials known for their unique optical and electronic properties. They observed intricate details about exciton recombination and diffusion processes in monolayer and bilayer MoS2, revealing distinct dynamics near crystal interfaces and in regions with nanoscale strain.
Extending their investigations, the researchers also examined vanadium dioxide (VO2), a material celebrated for its remarkable phase-change properties. Using their advanced imaging techniques, they mapped out the nanoscale distribution of metallic and insulating phases in bent VO2 nanobeams.
"We've been able to directly image the coexistence of different phases in VO2 with unprecedented detail," says Yang. "This allows us to understand how strain influences the material's electronic properties at a fundamental level."
Surprisingly, the team observed slower carrier recombination but faster diffusion in the metallic phase of VO2 compared to its insulating phase. This finding provides new insights into the material's behavior during phase transitions, which could be crucial for developing advanced switching and memory devices.
The research also highlighted the impact of local material properties, such as strain and interfaces, on exciton and carrier dynamics in both TMDCs and VO2. This understanding is vital for engineering devices that can leverage these nanoscale effects for improved performance.
Prof. Grigoropoulos said, "These techniques open up new possibilities for studying a wide range of nanomaterials and nanodevices. We're excited about the potential applications in fields ranging from energy harvesting to quantum information processing."
The combined findings from these studies demonstrate the power of advanced nanoscale imaging techniques in unraveling the complex physics of nanomaterials. As researchers continue to refine these methods, we can expect further breakthroughs in our understanding of materials at the atomic scale, paving the way for innovative technologies that harness the unique properties of nanomaterials.
These discoveries have significant implications for the development of next-generation electronic and optoelectronic devices, including high-performance sensors, memory devices, and adaptive optical components.
The ability to probe and manipulate material properties at such fine scales promises to accelerate the development of more efficient and capable technologies across a broad spectrum of applications.