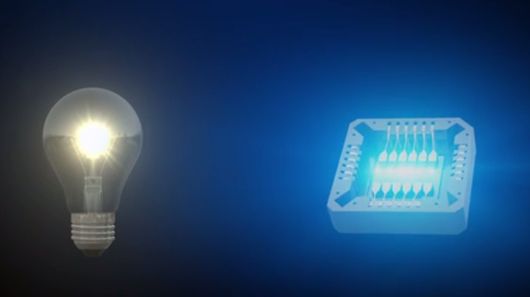
Over 130 years ago, Thomas Edison used carbon as the conducting filament in the very first commercial light bulb. Now a team of scientists and engineers have used that very same element, in its perfectly crystalline form of graphene, to create what they claim is the world's thinnest light-bulb. Even though just one atom thick and covering an area almost too small to see unaided, the new device is so bright that the light it produces can easily be seen with the naked eye.
Over 130 years ago, Thomas Edison used carbon as the conducting filament in the very first commercial light bulb. Now a team of scientists and engineers have used that very same element, in its perfectly crystalline form of graphene, to create what they claim is the world's thinnest light-bulb. Even though just one atom thick and covering an area almost too small to see unaided, the new device is so bright that the light it produces can easily be seen with the naked eye.
Researchers and engineers from Columbia University, Seoul National University (SNU), and Korea Research Institute of Standards and Science (KRISS) created the device using tiny filaments of graphene attached to metal electrodes, with these strips then suspended above a silicon substrate. Passing current through the filaments caused them to heat up to over 2,500° C (4,500° F) and produce an exceptionally bright light.
"We've created what is essentially the world's thinnest light bulb," said James Hone, Professor of Mechanical Engineering at Columbia. "This new type of 'broadband' light emitter can be integrated into chips and will pave the way towards the realization of atomically thin, flexible, and transparent displays, and graphene-based on-chip optical communications."
Interestingly, the ability of graphene to reach such elevated temperatures without melting either the underlying substrate or the metal electrodes is because, as graphene is heated up, it is less able to conduct heat away from itself. As a result, the concentration of heat is limited to the very center of the filaments and an exceptionally intense light is produced.
Measuring the spectrum of light emitted from the new device, the researchers also discovered that it peaked at wavelengths not expected to be seen. This was a result of interference between the light being generated directly by the glowing filaments and light bouncing off the silicon substrate and back through the graphene filaments themselves.
"This (phenomenon) is only possible because graphene is transparent, unlike any conventional filament, and allows us to tune the emission spectrum by changing the distance to the substrate."
A graphene lattice is also a particularly efficient way to produce light, due to its inherent ability to maintain excitation levels that allow the freer flow of electrons. That is, just as graphene is able to rapidly emit electrons when excited by lasers as the electrons remain at an elevated state, it also provides a similar capability for efficient photon release in an electrically-heated situation.
"At the highest temperatures, the electron temperature is much higher than that of acoustic vibrational modes of the graphene lattice, so that less energy is needed to attain temperatures needed for visible light emission," said Myung-Ho Bae, a senior researcher at KRISS. "These unique thermal properties allow us to heat the suspended graphene up to half of the temperature of the sun, and improve efficiency 1,000 times, as compared to graphene on a solid substrate."
Not the first graphene light-bulb – University of Manchester researchers lay claim to that – but certainly the thinnest, the new device also opens up many possibilities of alternative light generation at the microscale, particularly for on-chip generation for use in photonics devices.
As such, the researchers are currently working on ways to improve the performance of these incandescent devices in finding out how quickly they might be toggled on and off so that they may be used to generate ones and zeroes in optical communication. They are also exploring methods for incorporating them into flexible materials.
"We are just starting to dream about other uses for these structures – for example, as micro-hotplates that can be heated to thousands of degrees in a fraction of a second to study high-temperature chemical reactions or catalysis." said Professor Hone.